The Fraud of the Light Perception and its Implications on Chameleon Color Change Understanding and Lighting Choice
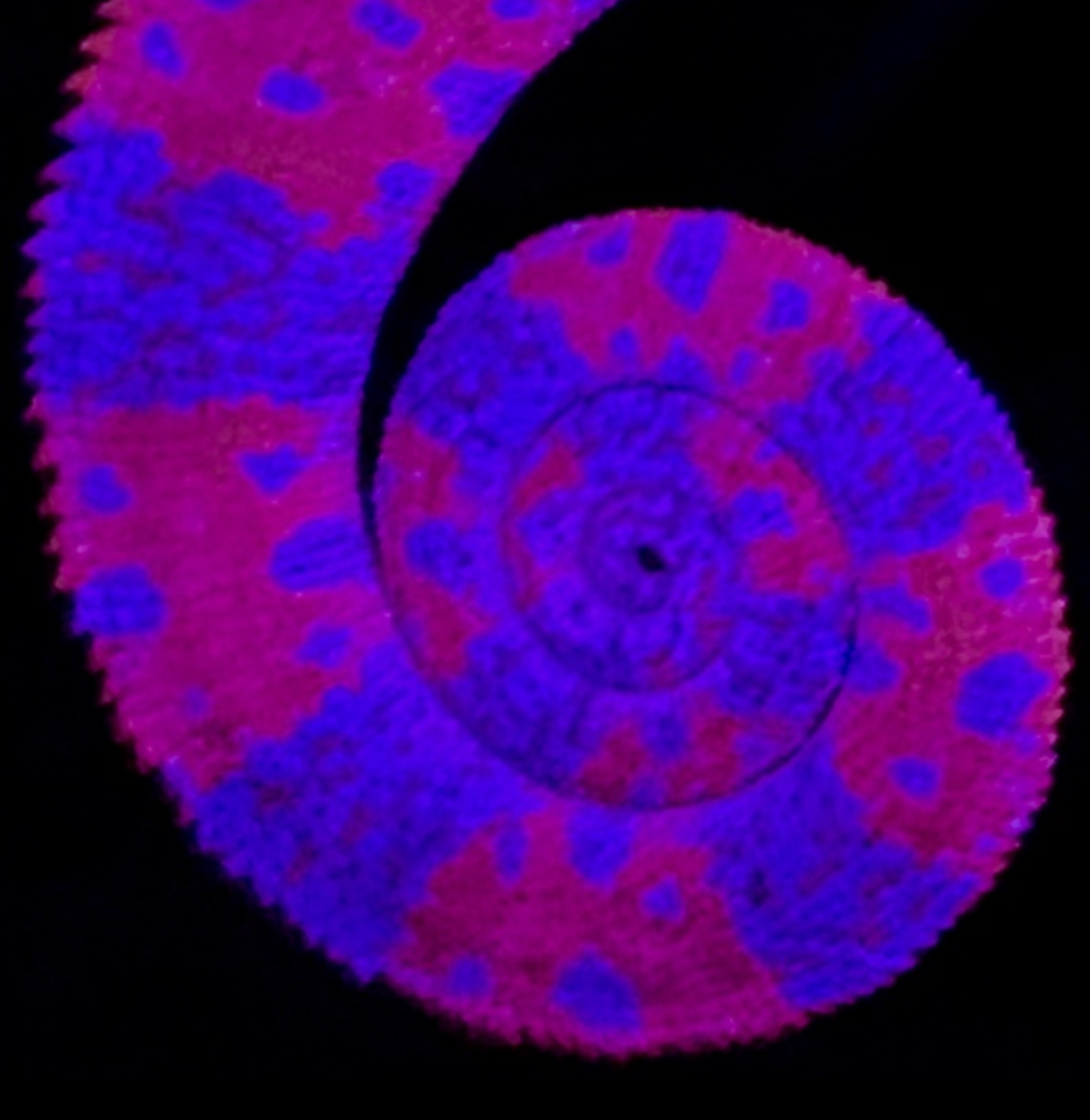
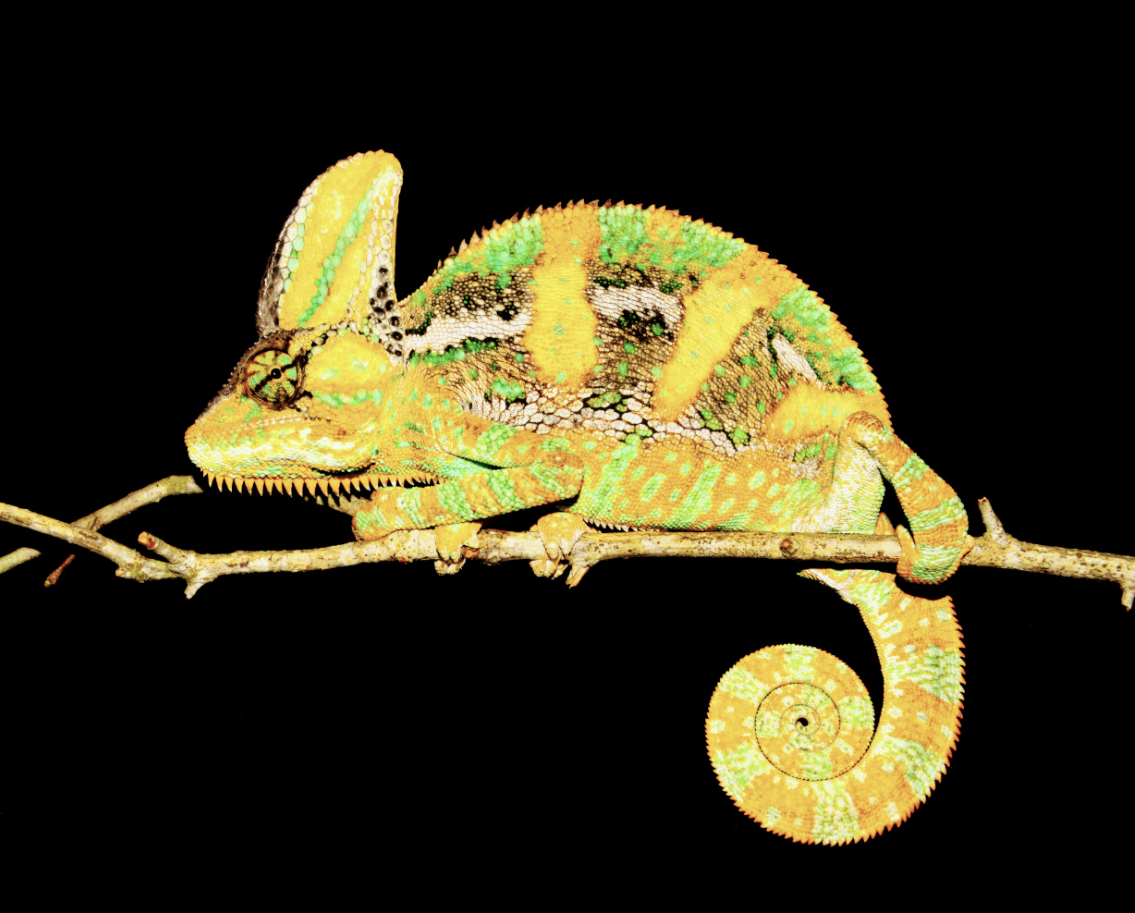
The Fraud of the Light Perception and its Implications on Chameleon Color Change Understanding and Lighting Choice
aka
Chameleons Shine But Get Blindfolded by Lamps
Chameleons are masters of many wonders of Mother Nature. There is a specific way they work with light throughout the day and even night and put on an impressive display. Before we elucidate that magical phenomenon, let us delve into the field of physics.
THE MAGIC OF (IN)VISIBLE) LIGHT
First, let us define what light is and what its composition entails.
Light is a form of electromagnetic radiation that can be perceived by the human eye. It is a stream of particles known as photons and has properties of both waves and particles, a characteristic termed wave-particle duality. The electromagnetic spectrum encompasses a wide range of electromagnetic radiation, including wavelengths that are both visible and invisible to humans.
Electromagnetic Spectrum Overview:
The electromagnetic spectrum is the range of all types of electromagnetic radiation, classified by wavelength and frequency.
1. Ultraviolet (UV) Radiation:
Wavelength Range:Approximately 10 nm to 400 nm.
Description: UV radiation is invisible to the human eye and can be divided into three categories: UVA (320-400 nm), UVB (280-320 nm), and UVC (100-280 nm). UV rays are known for their ability to cause sunburn and are used in sterilization processes due to their germicidal properties.
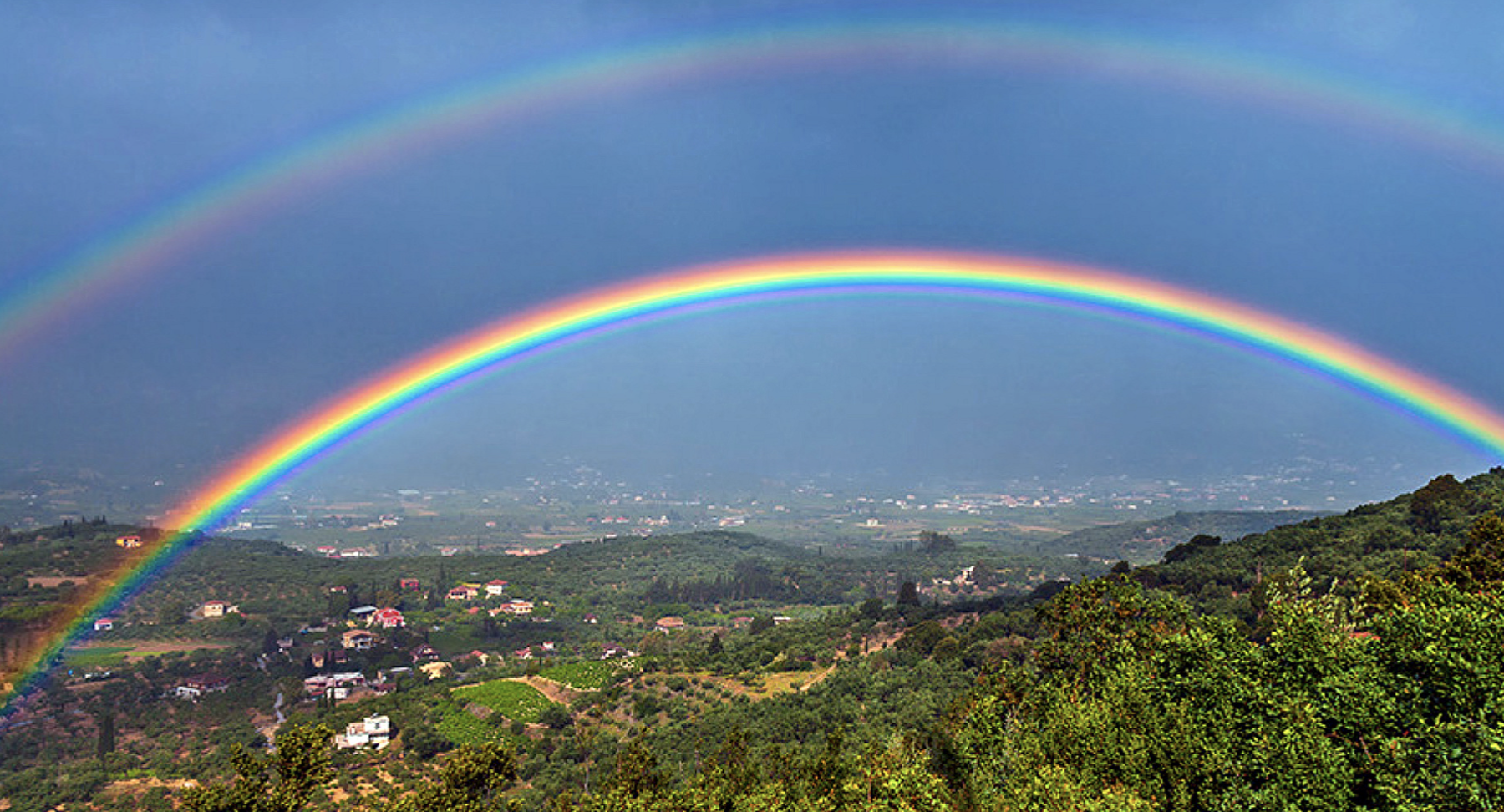

2. Visible Light:
Wavelength Range: Approximately 400 nm (violet) to 700 nm (red).
Description: This range includes all the colors of the rainbow: Violet (400 nm), Blue (450 nm), Green (500 nm), Yellow (570 nm), Orange (590 nm), Red (620-700 nm). These colors represent the wavelengths that can be seen by the average human eye, with each color exhibiting distinct properties within this spectrum.
3. Infrared (IR) Radiation:
Wavelength Range: Approximately 700 nm to 1 mm.
Description: Infrared radiation is also invisible to the human eye and is primarily associated with heat. It is often utilized in various technologies, including thermal imaging, remote sensing, and night vision devices.
By understanding both visible light and the broader electromagnetic spectrum, we can gain insight into how light interacts with matter and its various applications in science and technology.
CHAMELEONS SEE MORE
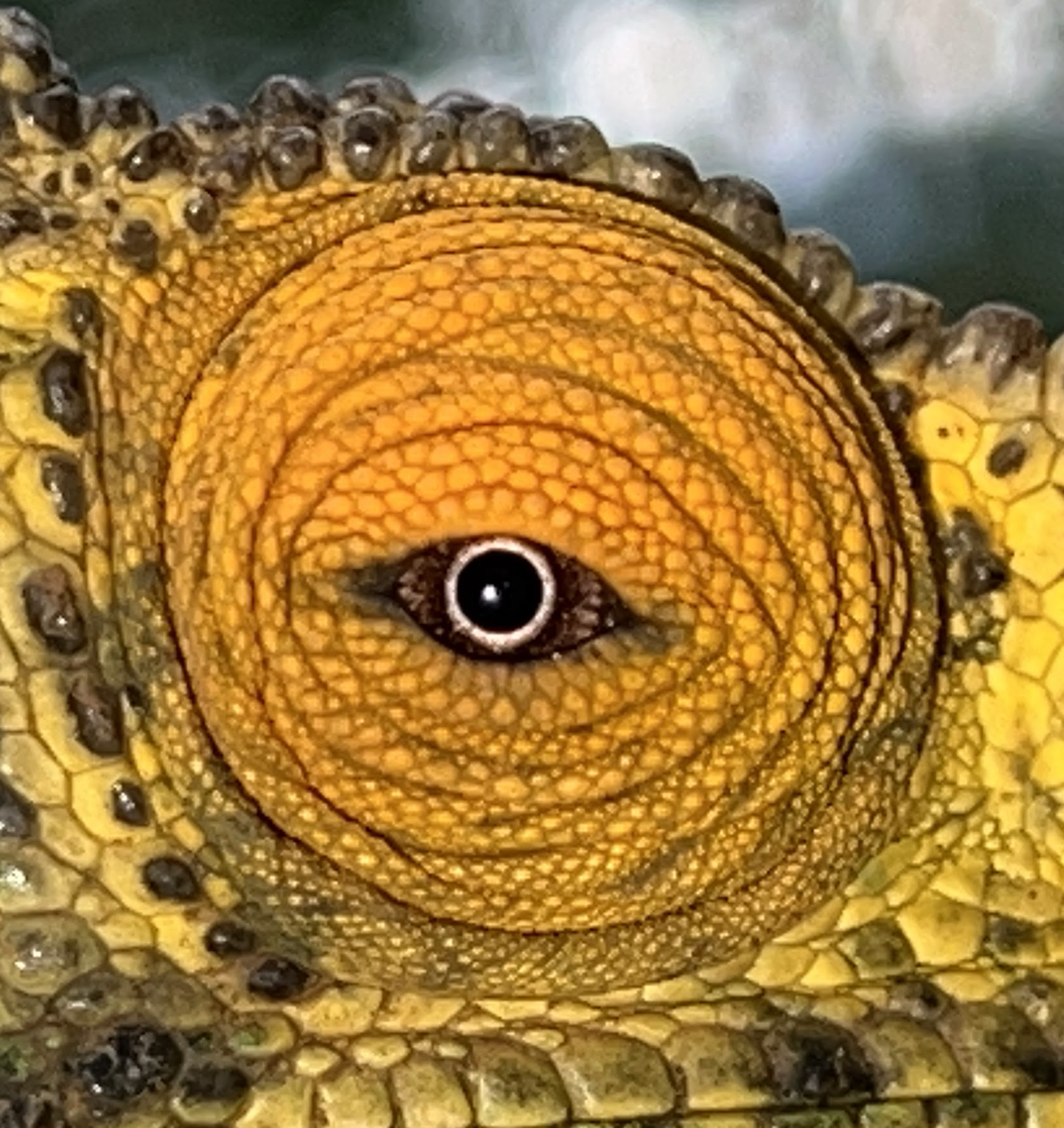
Chameleons have been shown to perceive a broader spectrum of colors in their environment compared to humans. While humans can detect wavelengths of light in the range of approximately 400 nm (violet) to 700 nm (red), chameleons possess an advanced visual system that allows them to see ultraviolet (UV) light as well. This ability enables them to perceive wavelengths below 400 nm, giving them access to a color spectrum that includes hues and patterns invisible to the human eye.
This enhanced color perception plays a crucial role in their behavior, helping them with tasks such as finding food, avoiding predators, and communicating with other chameleons. The ability to detect a wider variety of colors allows chameleons to better navigate their complex environments and utilize their remarkable color-changing abilities effectively.
THE VISION
Ths next issue we need to understand is how the colors of any objects are perceived. To do this, we must begin with the physiology of vision.
Physiology of Vision:
Vision begins when light enters the eye through the cornea and is focused by the lens onto the retina, the light-sensitive layer at the back of the eye. The retina contains two types of photoreceptor cells: rods and cones.
1. Rods:
Rods are responsible for vision in low light conditions and do not detect color. They are highly sensitive to light and allow us to see in dim environments, but because they do not respond to different wavelengths of light, they do not contribute to our perception of color.
2. Cones:
- Cones are the photoreceptor cells responsible for color vision. There are three types of cones, each sensitive to different ranges of wavelengths corresponding to different colors:
S-cones (Short-wavelength): Sensitive to blue light (approximately 400-500 nm).
M-cones (Medium-wavelength): Sensitive to green light (approximately 500-600 nm).
L-cones (Long-wavelength): Sensitive to red light (approximately 600-700 nm).
The brain processes signals from these cones, allowing us to perceive a wide spectrum of colors through the combination of inputs from different types of cones.
In summary, the combined activity of rods and cones in the retina, along with the brain's interpretation of these signals, creates our perception of color. Understanding this physiological process is essential for comprehending how organisms, including chameleons, perceive their environment and interact with it.
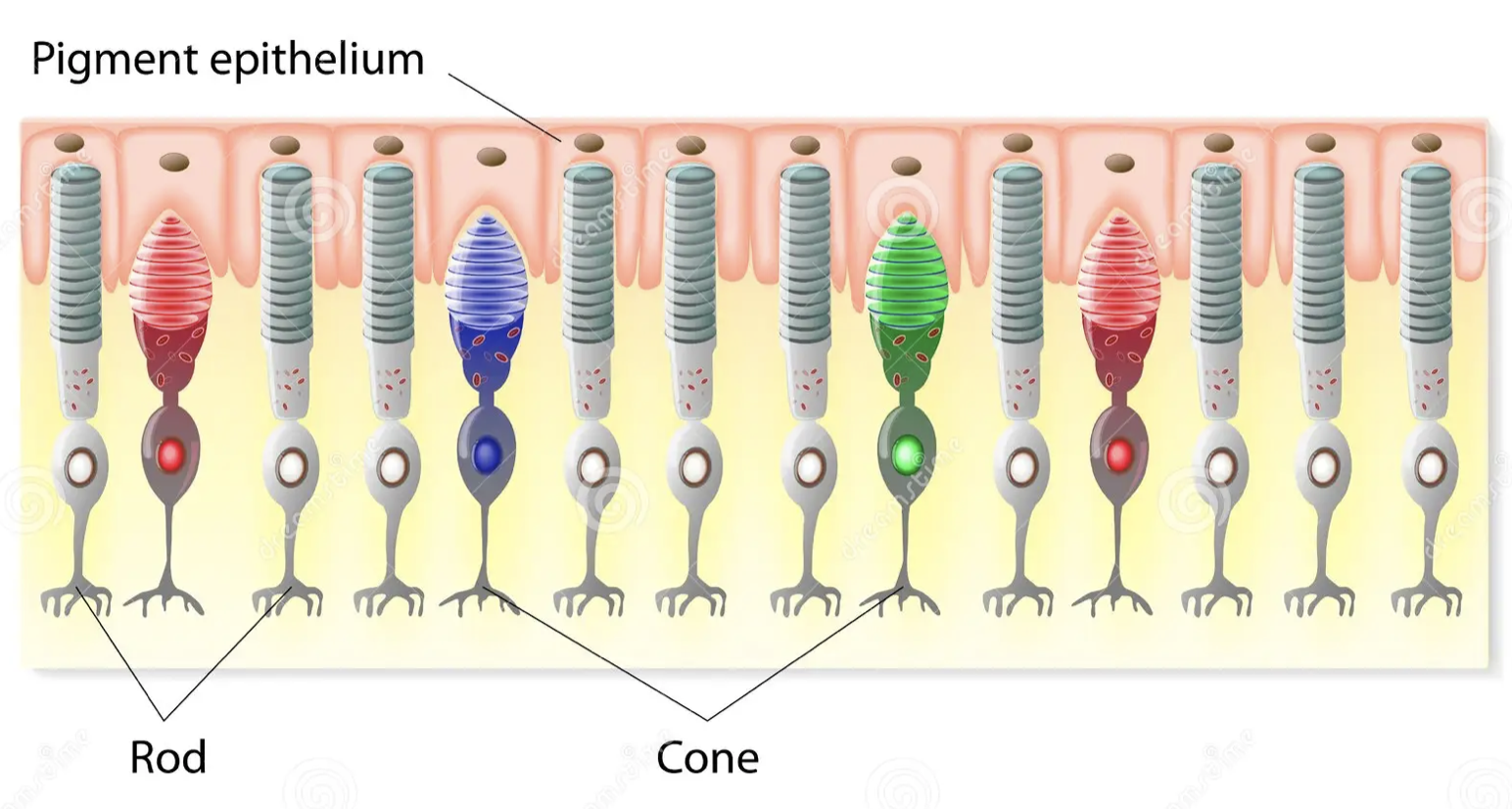
FRAUD IN COLOR PERCEPTION
The next part of the puzzle is the fraud in color perception. The color of any object is, in essence, a complex interplay of light and perception rather than a direct reflection of its inherent qualities. This concept is somewhat tricky, as it involves understanding the nature of light, absorption, and reflection.
When we look at an object, what we perceive as its color is determined by the wavelengths of light that are reflected off its surface and into our eyes. For instance, if an object appears red, it is because it reflects wavelengths of light that correspond to red (approximately 620-750 nanometers) while absorbing all other wavelengths in the visible spectrum. In this sense, the red object is not truly "red" in a qualitative way; instead, it can be understood as a selective filter that interacts with light.
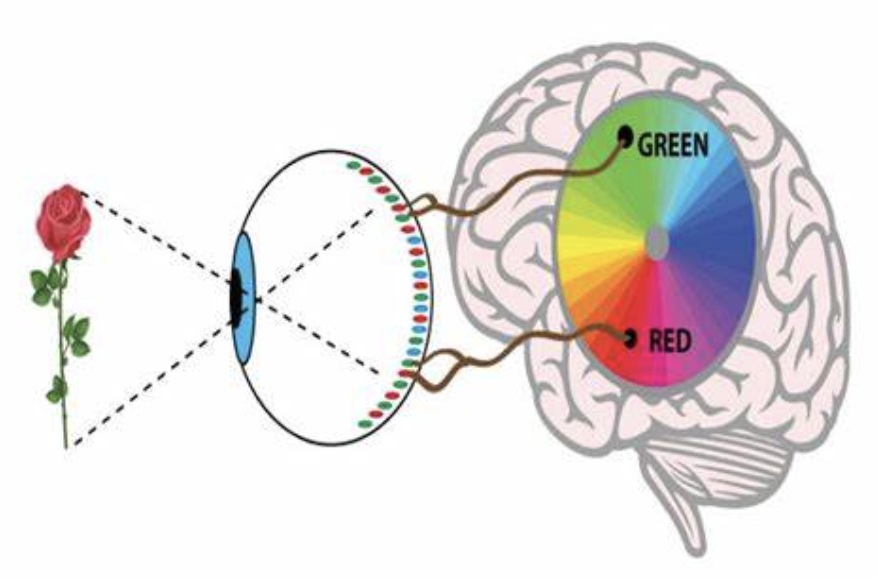
This occurs because of the material properties of the object. Every color corresponds to a specific range of wavelengths, and materials have molecular structures that determine which wavelengths they absorb and which they reflect. For example, a green leaf appears green because it absorbs red and blue wavelengths, reflecting primarily green wavelengths.
Our eyes, equipped with cones sensitive to different wavelengths, interpret the reflected light. This is where our perception becomes intriguing. The reflection of particular wavelengths is what our brain interprets as color. Thus, we can be "fooled" into believing that an object has a specific color due to the light it reflects, even though the true nature of that object is far more complex.
Additionally, the perception of color can change under different lighting conditions. The presence of other colors can influence how we perceive a particular color, a phenomenon known as color constancy. Our brains use contextual clues to maintain a consistent perception of color despite varying lighting conditions.
In summary, what we perceive as color is not merely a fixed attribute of an object but rather the result of intricate interactions between light waves and the physical properties of materials. The reflected wavelengths create the illusion that an object possesses a particular color when, in reality, it is engaging in a complex dialogue with light. This understanding underscores the fascinating relationship between light, materials, and perception, mirroring some of the complexities seen in the color-changing abilities of chameleons.
CHAMELEON COLOR CHANGE PHYSIOLOGY
Recent studies have provided a modern understanding of the physiology of color change in chameleons, emphasizing the role of structural colors rather than solely relying on pigment changes as traditionally believed. This shift in perspective highlights the sophistication of chameleons' color-changing abilities, particularly focusing on the refraction of light through specialized layers of guanine crystals in their skin.
Physiology of Color Change in Chameleons:
Chameleons are renowned for their striking ability to change color, a phenomenon once thought to be primarily due to the modulation of pigments, similar to how melanin operates in melanocytes. However, contemporary research has revealed that their color change is significantly influenced by the physical arrangement and structural properties of reflective cells, specifically the layers of guanine crystals in their skin.
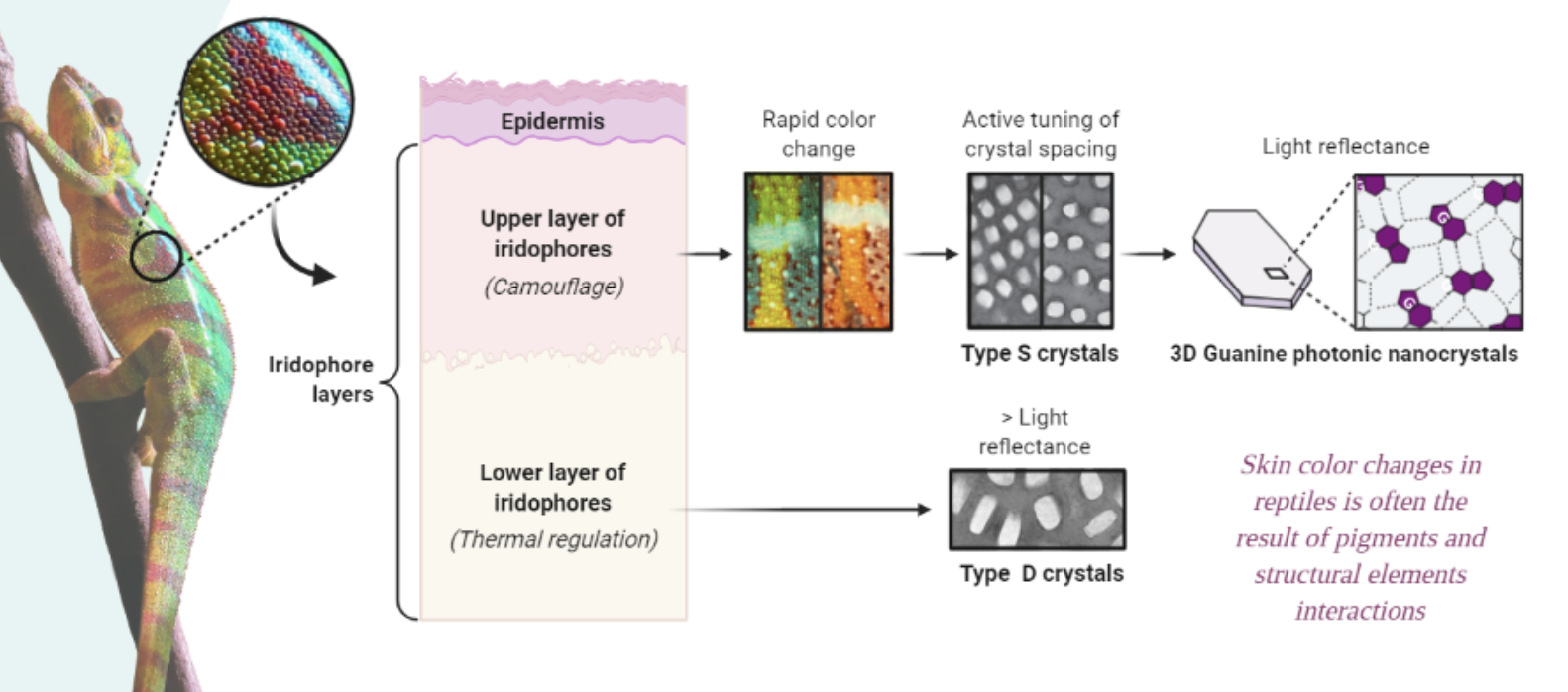
Guanine Crystals and Structural Colors:
The skin of chameleons contains unique structures known as iridophores, which are cells filled with guanine crystals. These crystals are arranged in layered patterns that can refract and reflect light in various ways. When light strikes the chameleon's skin, it interacts with these crystal layers, leading to a variety of visual effects. The geometry and spacing of the layers can change in response to neural and hormonal signals, allowing the chameleon to manipulate the wavelengths of light that are reflected.
1. Refraction of Light:
As light enters the skin, it passes through the layers of guanine crystals. Due to the differences in refractive indices between the crystals and the surrounding medium, some wavelengths of light are reflected while others are absorbed. This selective reflection creates structural colors that can appear significantly different depending on the angle of light and the observer's perspective.
2. Dynamic Color Change:
By altering the spacing and arrangement of the guanine layers in response to stimuli (such as temperature, mood, or social interactions), chameleons can effectively change the color they display. This dynamic process enables them to blend into their environments for camouflage, communicate with conspecifics, or regulate their body temperature.
Contrast with Traditional Beliefs:
This modern understanding challenges the traditional notion that chameleon color change is predominantly attributable to pigment granules, such as those found in melanin-containing cells. While pigments still play a role, the structural coloration provided by guanine crystals is a significant and often more effective mechanism for achieving vibrant and varied colorations.
The contemporary view on the physiology of color change in chameleons emphasizes the crucial role of light refraction through guanine crystal layers in their skin. This represents a remarkable adaptation that goes beyond mere pigment changes, illustrating the complexity and sophistication of chameleon coloration as an evolutionary trait.
CHAMELEONS GLOW AND SHINE
Whoever undertakes the same journey of finding chameleons in the wild at night will share the same impressions as I do, having spent countless nights searching for and discovering these remarkable creatures in the bushes and trees of Madagascar, diverse African territories, India, and the Hawaiian Islands. I've even explored parts of California and Florida, almost forgetting my home in Europe, where I also conducted my studies of chameleons.
The magical and primordial hunt for chameleons at night can be filled with frustrations, as they are not as easy to spot as it might seem. However, once you locate the first one, your chances of finding others increase significantly. While sharing this adventurous and mystical activity with colleagues and friends, we have often been astonished by how chameleons stand out from the surrounding vegetation, almost as if they possess a light of their own.
In fact, they do shine, and this is due to the previously explained color change mechanisms. Chameleons allow the full spectrum of light to enter their skin, which is then reflected off guanine crystals located beneath the surface. This process results in only one specific color being reflected back towards us, while all other wavelengths are absorbed by the skin.
Thus, chameleons can be likened to small lamps in their environment. They capture light rays and photons from sources like our flashlights and reflect them back, creating the illusion that they glow. Witnessing this captivating phenomenon, chameleons shimmer and shine like Christmas trees in the darkness, enchanting anyone fortunate enough to experience this spectacle.
CHAMELEONS GLOW INVISIBLY FOR HUMANS TOO
Modern studies by my friend and colleague David Protzell from Germany reveal an even more spectacular phenomenon: chameleons are capable of fluorescence. This ability allows them to absorb ultraviolet (UV) light and re-emit it at longer wavelengths, effectively "glowing" in the UV spectrum, which is invisible to the naked eye. When exposed to blacklight, chameleons can exhibit vibrant colors that are not detectable under normal lighting conditions.
In this case, however, it is not the skin itself that actively participates in this process, but rather their bones and bone processes, laying shallowly underneath the skin surface or even coming to the sirface itself. The fluorescence originates in the bones, which lie beneath the skin, specifically underneath the keratinous layers. These bone structures interact with UV light and transform it into visible light. This remarkable capability enhances their visual appearance and serves functions in communication and mate attraction while providing camouflage in their environment. The intricate interplay between their bones and light adds another layer of complexity to the chameleons' dynamic visual experience in the dark. we need to rewrite lits of chapters of chameleon biolgy due to these new findings. They are not as strictly diurnal as we believed for centuries.
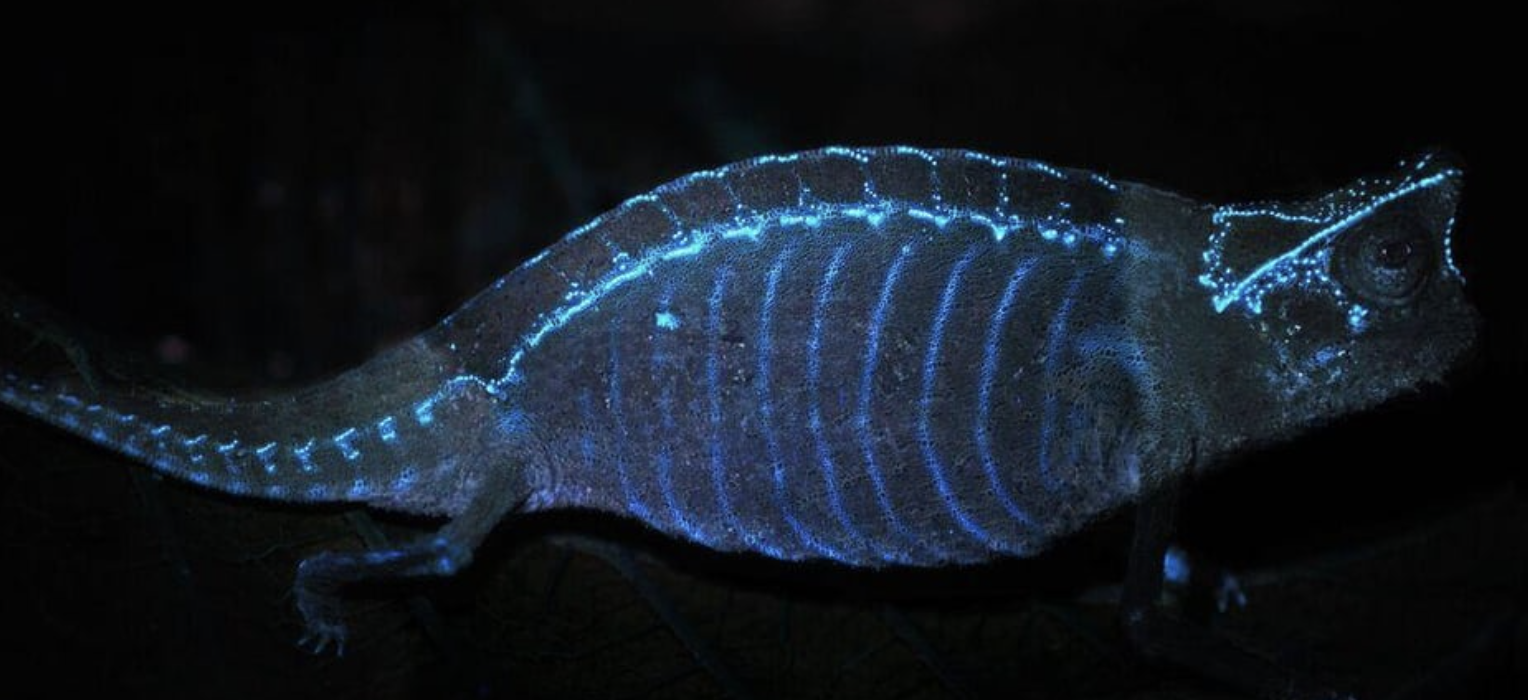
Practical implications
What are the implications of all the above-mentioned pieces of information for chameleons in the wild and in human care? There are at least two major areas which I'll take out from the above-mentioned facts.
UNDERSTANDING OF BEING FOULED
We need to understand that chameleons can actually mislead us with their colors, acting almost like a photographic negative of reality. Our eyes are designed in a way that they can sometimes deceive us, allowing chameleons to manipulate our perception and make us believe what they want us to believe. Additionally, it is crucial to recognize that the colors chameleons reflect depend on the spectrum of light we shine on them.
Imagine, for instance, a chameleon that appears completely red illuminated solely by red light beams at night. In such a scenario, the chameleon would blend seamlessly into its surroundings or appear nearly indistinguishable from the light source, effectively camouflaging itself. Since it reflects the same wavelengths of light as those it receives, it would not stand out but rather would vanish into the environment created by the red illumination. This highlights how a chameleon's color change is not just about its inherent traits, but also about interactions with external light sources, shaping our perception of reality.
If we were to illuminate a completely red chameleon with light beams from which the red spectrum has been filtered out, leaving only the other colors, the chameleon would appear quite different than its usual vibrant red.
In this case, since the chameleon cannot reflect red light—because there is none in the environment—it would not be able to display its red coloration. Instead, it would likely reflect other available wavelengths, such as green, blue, or yellow, depending on the pigments and structural features present in its skin. Consequently, the chameleon might appear dull or could take on a completely different color, such as a muted green or brown, rather than maintaining its striking red, maybe even turn black or - who knows - become totally invisible…
This scenario highlights how a chameleon's coloration is not solely an intrinsic characteristic but is also heavily influenced by the surrounding light conditions. The interaction between the chameleon's pigmentation and the light spectrum available significantly shapes its observed color, demonstrating the complexities of color perception and camouflage in these fascinating creatures.
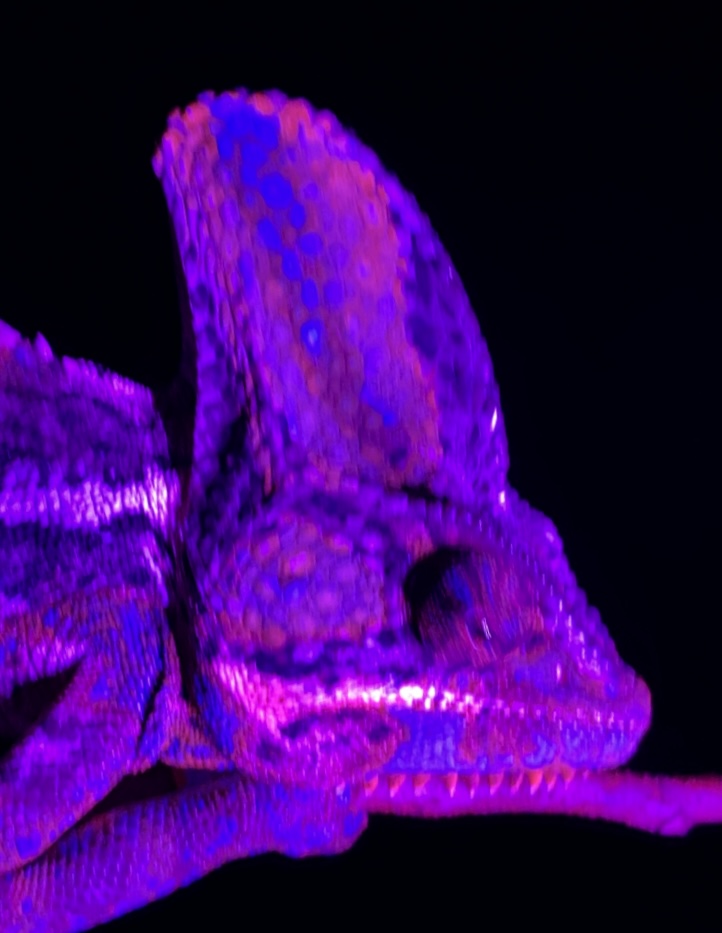
A complete transformation occurs when we use a torch fitted with blacklight on a colorful Yemen chameleon. Under blacklight, which emits ultraviolet (UV) light, the chameleon's ability to fluoresce becomes prominent.
In normal lighting, the Yemen chameleon displays its vibrant colors, including greens, blues, yellows, and oranges. However, when subjected to blacklight, the chameleon may exhibit even more vivid and unexpected colors due to its fluorescence. The absence of specific light spectra means that the chameleon's colors can appear dramatically enhanced or altered, revealing hues that are typically invisible under regular lighting conditions.
Moreover, the chameleon's skin may shimmer with patterns that are difficult to see in standard light. This interaction between the Yemen chameleon's skin and UV light underscores the complexity of its coloration and demonstrates how external lighting can significantly change our perception of these captivating creatures. Using a blacklight unveils an extraordinary spectacle, showcasing the chameleon's hidden beauty and adaptability in an astonishing way.
Coloration of a chameleon if exposed to various color spectrum composition
ILLUMIONATION OF CAGES
The second implication relates to the usage of light for the illumination of chameleon cages. Recently, lamps with modified spectra—such as red, pinkish grow lights for plants, or even blue lamps—have been introduced into chameleon husbandry. However, their usage is highly problematic and strongly discouraged. These colored lights can alter the perception of the surroundings for chameleons in an unnatural way, causing objects and habitats to appear in completely different colors or even making some objects seemingly disappear altogether.
Such alterations can lead to significant stress and confusion for chameleons, negatively impacting their overall well-being and health. Therefore, the principles of naturalistic chameleon husbandry should be strictly followed. It is essential to imitate the chameleon's natural living conditions as closely as possible, including the spectral quality of light.
Using any artificial light source at night, including heat emitters, is indeed considered a significant concern in chameleon husbandry. In the wild, chameleons are primarily accustomed to moonlight, which is a very low-intensity light, typically around 0.01 lux. This natural light level provides cues for their nocturnal behaviors and rhythms.
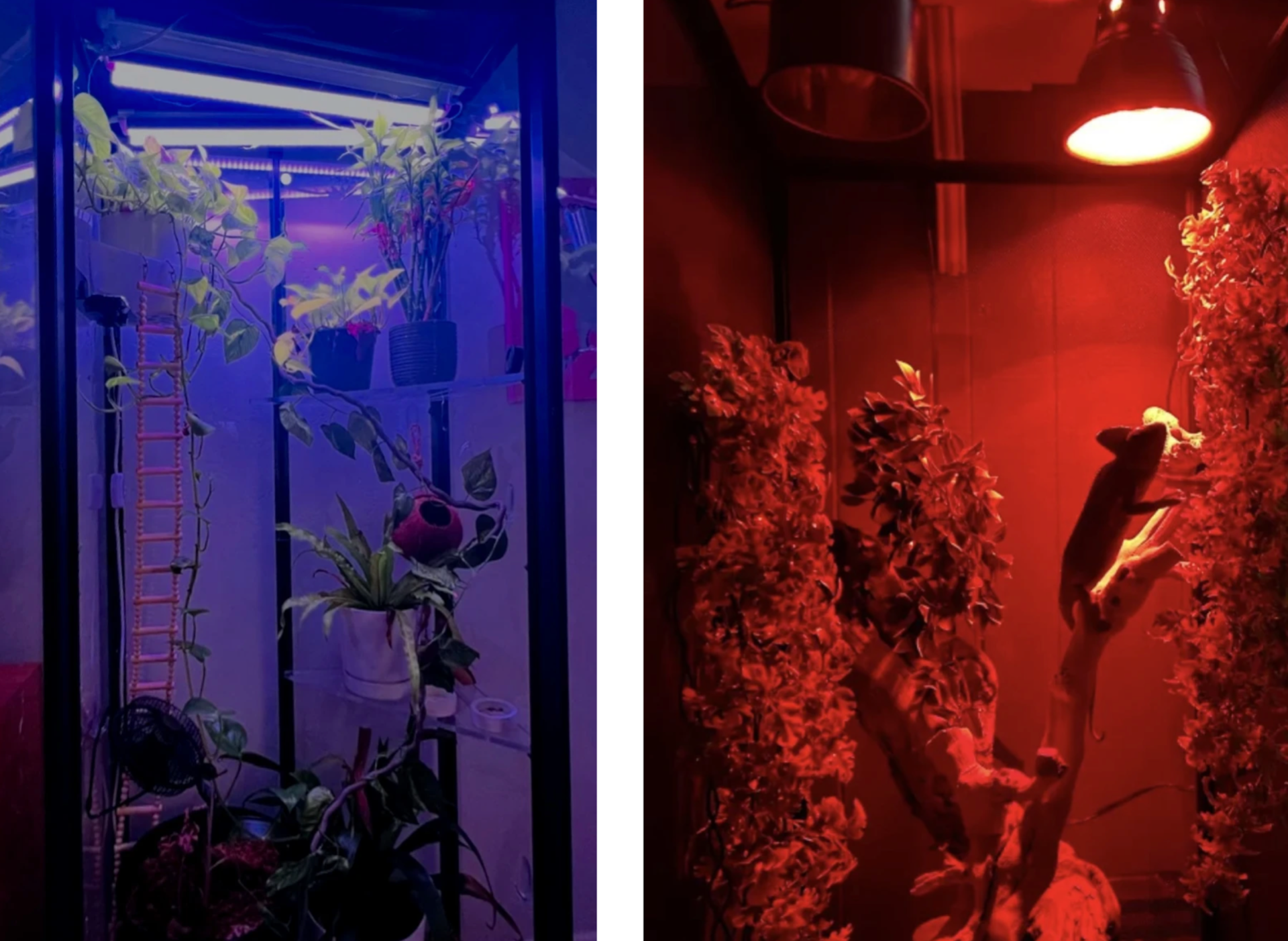
Any other forms of artificial light can be perceived by the parietal eye, also known as the "third eye," located on the top of the head between the two regular eyes. This organ, which is often covered by a thin layer of skin, can detect changes in light intensity and the presence of light, even if it is not in the visible spectrum. The exact function of the parietal eye and the connected pinneal organ is still being researched, but it is understood to play a role in regulating circadian rhythms and may influence physiological processes that can impact the chameleon's health. For instance, exposure to excessive or unnatural light, especially at night, can disrupt the chameleon's sleep patterns. This disruption can lead to increased stress, discomfort, and tiredness, negatively affecting their overall well-being. To ensure proper husbandry, it is essential to mimic natural light cycles closely, allowing for a period of darkness at night that properly simulates the conditions the chameleons would experience in their natural habitats.
Manufacturers and sellers of lamps for chameleon husbandry offer absolute scrutiny as unnatural and HARMFUL
The final words
Always use full-spectrum lights that closely resemble natural sunlight, avoiding any modifications that could confuse chameleons. Additionally, it's important to recognize that chameleons can see both ultraviolet and infrared light. Incorporating a spectrum that includes UV light can enhance their environment and promote healthier behaviors. By prioritizing naturalistic lighting conditions, we can better support the health and welfare of chameleons in human care.
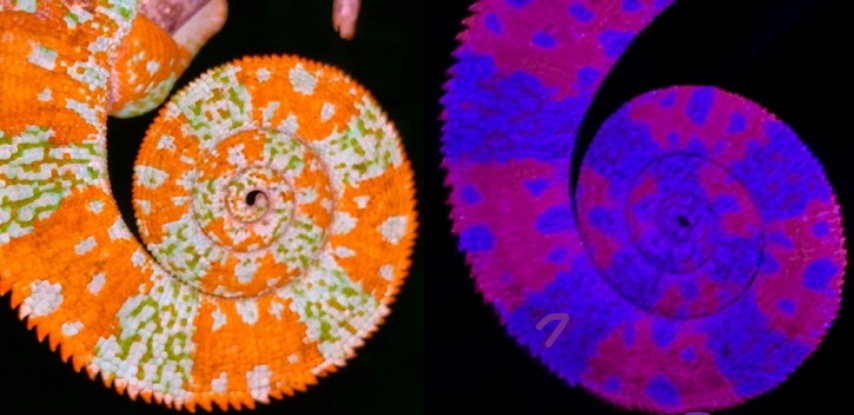